2020 PFAS Update Series, Part III
Review of membrane separation and ion exchange processes to treat contact water/leachate or groundwater to remove PFAS chemicals.
Part III
Craig Coker
Treating contact water/leachate or groundwater to remove PFAS chemicals is possible. EPA has assembled the Drinking Water Treatability Database, which can be used to identify effective drinking water treatment processes. As noted in Part II, the following processes were found to be effective for removal of PFOS: GAC (up to >99% removal in bench tests), high-pressure membranes such as nanofiltration and reverse osmosis (up to >99% removal), ion exchange (up to >99% removal), and powdered activated carbon (up to 99% removal).
Part II of this series reported on GAC. Part III examines membrane separation and ion exchange systems.
Membrane Separation
Membrane separation processes include nanofiltration (NF) and reverse osmosis (RO).
Membrane separation technologies produce clean water (called permeate) and a concentrated reject stream (called concentrate). RO separates compounds from water solutions by passing pressurized water across a semipermeable membrane. The feed pressure forces the water through the membrane against the natural osmotic gradient, thereby increasing the dissolved contaminant concentrations on one side of the membrane and increasing the volume of water with lower concentrations of dissolved contaminants on the other.
As the desired level of removal increases, the feed pressure also generally increases. For RO membranes, typical feed pressures range between 125 to 300 pounds per square inch (psi) for low pressure systems, 350 to 600 psi for standard pressure systems and 800 to 1,200 psi for seawater applications (EPA, 2020).
The molecular weight cut-off (MWCO) is a measure of the removal characteristics of a membrane (expressed in Daltons, a measurement unit of molar mass; 1 Dalton = 1.66 x 10-27 kilograms) in terms of atomic weight. The typical range of MWCO levels is generally less than 100 Daltons for RO membranes. Permeate passes through the membrane and the concentrate is collected for disposal or discharge, depending on the nature of the compounds and particles present.
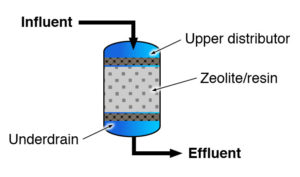
Figure 1. Reverse osmosis membrane
RO membrane systems frequently require some type of pretreatment to: 1) Condition the water for optimum membrane effectiveness; 2) Modify the feed water to prevent membrane fouling and plugging; 3) Maximize the time between cleanings and prolong membrane life.
The type of pretreatment required depends on the feed water quality and membrane type. The feed water must be generally free of suspended matter for RO systems. For this reason, storm water runoff may require more extensive pretreatment than groundwater. Turbidity and the silt density index (SDI) are typically used as indicators of feed water suspended solids. Acid and/or antiscalant addition is commonly used when scaling is the primary fouling concern. Scaling occurs when the concentrations of dissolved contaminants in the concentrate exceed the solubility product of a particular compound.
PFAS removal efficiencies of better than 99% have been shown, but the concentrate rejected by RO must be disposed of appropriately. It is possible that concentrate will not be accepted by a publicly owned wastewater treatment plant. One study at a 10.5 million gallons/day water reclamation plant in Queensland, Australia measured PFOA and PFOS concentrations before and after RO (Thompson, 2011), as noted in Table 1. The RO membranes at this plant are designed to filter out contaminants in the 100-300 Dalton (Da) range, and the Dalton values for PFOA and PFOS are 413 Da and 499 Da, respectively.
Ion Exchange
Ion exchange (IX) processes are reversible chemical reactions for removing dissolved ions from solution and replacing them with other similarly charged ions. IX processes can be either cationic (exchanging positively charged ions) or anionic (exchanging negatively charged ions). Water softening systems are examples of cation exchange. Anion exchange is used to remove contaminants such as nitrate, fluoride, sulfate and arsenic.
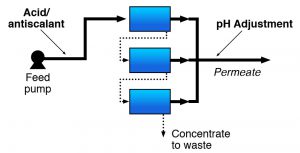
Figure 2. Ion Exchange. (mage courtesy of USEPA
The exchange medium consists of a solid phase of naturally occurring materials (zeolites) or a synthetic resin (small plastic beads) having a mobile ion attached to an immobile functional acid or base group. The mobile ions are exchanged with solute ions having a stronger affinity to the functional group (e.g., calcium ion replaces sodium ion or sulfate ion replaces chloride ion). The rate of removal is dependent on initial concentration of the contaminant, the concentration of competing ions, treatment design (i.e. flow rate, size of resin beads) and the nature of the ions within the beads (ITRC, 2008).
When the capacity of the resin is exhausted, it is necessary to regenerate the resin using a saturated solution to restore the capacity of the resin and return the resin to its initial condition. Brine, or sodium chloride solution, is most the commonly used regenerant, although others, such as strong acids (hydrochloric acid, sulfuric acid) or strong bases (sodium hydroxide) may also be used (EPA, 2020).
Anion exchange has been studied for its ability to remove PFOA, PFNA and PFOS. One publication reported on removal of PFOA, and PFOS at a New Jersey drinking water treatment plant using “porous anion exchange resin impregnated with iron oxide” to treat arsenic of 76% and >90%, respectively. In another study, two anion exchange treatments, not designed for PFC removal, demonstrated the effective removal of PFOA (74%), PFNA (>67%) and PFOS (>92%) (Cummings, 2015).
PFAS Removal At Composting Facility
One thing to remember about treatment technologies is demonstration of suitable performance at the intended scale. A review of the literature regarding treatment technologies for PFOA and PFOS indicates that much of the work is a laboratory bench-scale or pilot-scale. One of the more novel laboratory-scale approaches to treating PFAS in water is to use hemp protein (from hemp seeds) to absorb the PFAS (Tyree, 2019). However, in one composting facility design project the author is working on, planning is underway for PFAS removal from contact water using GAC treatment preceded by alum and polymer addition with suspended solids removal via a plate settler. The system would be sized for a continuous flow capacity of 50 gallons/minute (72,000 gallons/day) with a potential capital cost in excess of $1.5 million.
Craig Coker is a Senior Editor at BioCycle and CEO of Coker Composting & Consulting near Roanoke, VA.