B. Morelli, S. Cashman, C. Ma, J. Turgeon, and J. Garland
An in-depth environmental and economic assessment was conducted by the U.S. Environmental Protection Agency, in collaboration with Eastern Research Group, on food waste recycling options. This two-part article provides a summary of the study’s findings. A detailed technical presentation of this project’s methods, assumptions and results is available in a publicly accessible EPA report published in 2019 (Morelli, Cashman, et al., 2019). An appendix table, included with Part I , summarizes the most essential assumptions used to generate study results.
The scope of the research, described in Part I, is as follows:
- Compares the environmental and economic impact of recycling 1 kilogram (2.2 lbs) of typical commercial food waste (e.g. fruit, vegetable, meal scraps) through either codigestion at a wastewater treatment facility or composting in windrows or aerated static piles.
- Recycling options are compared against common disposal pathways — landfill and waste-to-energy incineration — using life cycle assessment (LCA) and life cycle cost analysis (LCCA) methods.
- Impacts are quantified in eight environmental categories covering global and regional impacts as well as life cycle costs (reported as net present value).
- Pathways modeled are specific to Massachusetts but are applicable to other communities trying to determine environmentally preferable and cost-effective management option(s).
Part I provided detailed global warming and summary results for all environmental impacts assessed for five food waste recycling and disposal systems — anaerobic digestion (AD) of food waste and biosolids (codigestion) at a wastewater treatment facility (WWTF), windrow and aerated static pile composting (ASP), landfilling, and waste-to-energy (WTE) incineration. The WWTF used as the reference for AD of food waste (codigestion) is the Greater Lawrence Sanitary District (GLSD) in Lawrence, Massachusetts.
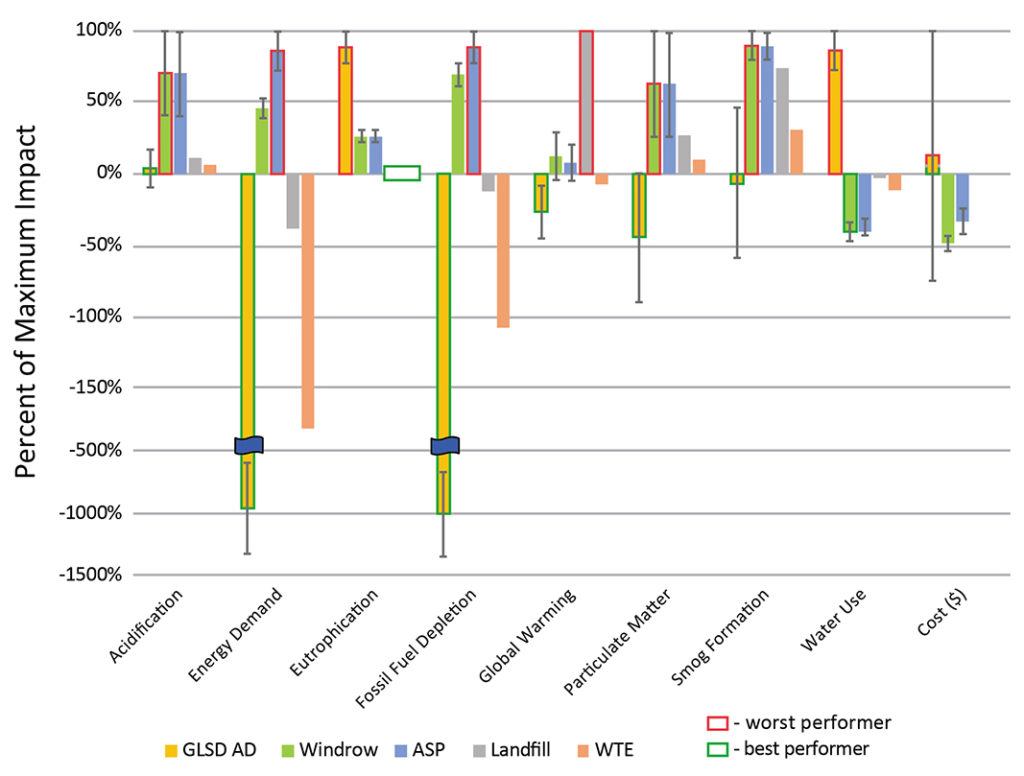
Figure 1. Summary LCA and cost results. Bar height represents average net impact potential for each treatment option as a percentage of maximum impact. Error bars mark high and low estimates of relative impact based on AD performance scenarios and compost process emission scenarios.
AD: anaerobic digestion, ASP: aerated static pile; WTE: waste-to-energy
Figure 1 provides a comparative summary. It also includes cost results for the three recycling options, but not for landfilling and WTE, which are no longer relevant disposal options for commercial food waste in Massachusetts.
Part II takes a closer look at the results for the following impacts: smog formation potential; eutrophication potential; energy demand and recovery; water use; acidification and particulate matter formation potential; and life cycle costs.
Smog Formation Potential
Hauling heavy food waste to recycling/disposal facilities emits sulfur dioxide. Life cycle analysis (LCA) quantifies the impact from these emissions as smog formation potential (SFP). LCA results confirm that transportation is a dominant contributor to SFP across all food waste treatment options. AD of food waste can yield a net negative SFP by replacing grid electricity with biogas-fueled CHP (combined heat and power). Composting options demonstrate the highest SFP due to the additional transport needed to get finished compost to the application site (compared to landfill) and no avoided energy production. Given the dependence of SFP results on transportation, results are expected to vary considerably depending upon regional hauling distances and the efficiency of fleet vehicles.
Eutrophication Potential
Eutrophication potential is an environmental impact category related to nutrient pollution of freshwater and marine water systems. Common sources of nutrient pollution include WWTFs and agricultural runoff, both of which relate directly to the end products of the two food waste recycling options. Our results showed that both AD and composting have higher eutrophication potential than landfill and WTE disposal options. Nitrogen and phosphorus, the main chemicals contributing to eutrophication potential, are both present in food waste. These nutrients are the basis of the avoided fertilizer production credit assigned both to finished compost and pelletized biosolids. Both the AD and composting recycling options keep these nutrients in active circulation, where they can both benefit agricultural production and impact water quality. Conversely, both landfills and WTE facilities take a large fraction of these nutrients out of active circulation.
Nutrient releases from the GLSD WWTF were estimated using GPS-X™, which was calibrated based on historical plant discharges. The GLSD WWTF does not have nutrient-based permit requirements. Considering eutrophication potential is especially important for ADs attached to WWTFs, where nutrient permit limits must be considered. Advanced wastewater treatment systems are available to remove nitrogen and phosphorus from effluent wastewater. But these treatment options have higher embedded energy and chemical demand than conventional treatment processes not intended for nutrient removal (Neethling and Kennedy, 2018).
Energy Demand and Recovery
Energy demand results highlight the energy recovery potential of food waste. AD, WTE and landfilling can all capture some of the available energy in food waste. AD is the most efficient means of energy capture. GLSD’s codigestion project is expected to make the WWTF self-sufficient in production of heat and electricity. In fact, a surplus of heat energy is produced and could be used for district heating or industrial applications. The LCA model only assigns environmental credits for recovered heat used onsite that avoids natural gas consumption. WTE combustion also yields a net surplus of energy, but produces considerably less energy than AD treatment, due largely to the high water content of food waste and the challenge that this poses for combustion.
Energy recovery credits from the landfill are modest, similar in magnitude to reductions in energy demand related to avoided fertilizer production for composting systems. Composting, though, has a net positive energy demand and fails to capture the energy content of food waste. Similar trends were observed for fossil fuel depletion potential.
Water Use
Composting has a net negative water consumption, due mainly to avoided fertilizer production. Water use is highest for AD treatment, due to assumptions related to water consumption during production of the engineered food waste bio-slurry brought to GLSD for codigestion. Food waste has about 30% solids and must be diluted before digestion. If other, higher liquid waste streams were blended with food waste, this water use would not be necessary and AD water use would also be net negative. As this study focuses on food waste disposal, water reuse at the WWTF was excluded from the analysis.
Acidification and Particulate Matter Formation Potential
Both of these environmental impact categories are related to air emissions. Observed trends for them are similar, with the GLSD AD treatment option producing environmental benefits through avoided grid electricity consumption and natural gas combustion. Composting can have widely varying results depending on process emissions, but it yields the highest relative impacts regardless of scenario assumptions. Compost piles can produce considerable ammonia emissions if not managed properly, which contributes to environmental impact in both categories. The ASP’s biofilter was only modeled as reducing methane emissions, however removal efficiencies of ammonia, which contributes considerably to impact in these categories, in excess of 0.96 have been reported (McNevin and Barford, 2000). Employing biofilters and implementation of other composting Best Management Practices could considerably reduce acidification and particulate matter formation potential impacts modeled here.
Life Cycle Costs
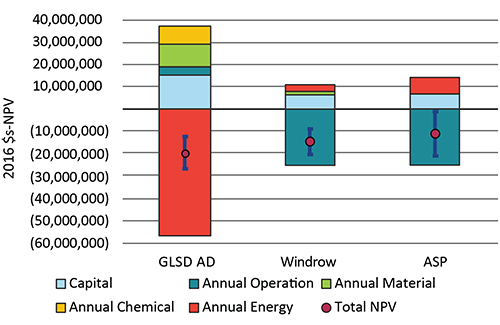
Figure 2. System net present value AD: anaerobic digestion, ASP: aerated static pile; NPV: net present value
Figure 2 presents system net present value (NPV) for the AD, windrow and ASP food waste recycling options. Presented results represent average life cycle costs over 30 years. NPV is negative across all three systems, indicating that revenues exceed expenditures over 30 years. Interestingly, there is considerable overlap in NPV among the three treatment options, with slightly more revenue potential from the AD system at the lower end of the projected cost range. Results for the AD process represent average performance results assuming full use of the digesters’ available capacity. Results presented in the EPA report (Morelli, Cashman, et al., 2019) show that at 50 percent of capacity, no payback period exists for the investment in AD codigestion capacity. The cost results in Figure 2 represent the potential cost range across the partial and full capacity scenarios and in both AD performance scenarios.
Costs for the windrow and ASP systems were based on relatively simple farm-based composting systems, which seem to predominate in Massachusetts. As it would take roughly 14 of these composting facilities to equal the food waste processing capacity of GLSD’s digesters, composting costs presented in this figure represent aggregate costs of operating 14 individual composting facilities. The AD process has considerably higher capital and operational costs, but these are accompanied by greater revenue potential from the sale of electricity, renewable energy credits (electricity) and alternative energy credits (heat). Tipping fees, included in operational costs, produce the bulk of the revenue for the composting systems.
Final Takeaways
Results of this study validate several common talking points on food waste disposal bans and other mandatory organics recycling laws, including the global warming potential benefit of avoiding landfill disposal of food waste. Smog formation potential results, shown in aggregated format in Figure 1, reflect transportation’s contribution to the generation of pollutants that can degrade local air quality, serving as a partial justification for the distance-based exemptions common among existing organic waste regulations.
Results also show AD’s potential to outperform composting, landfills and WTE facilities in most environmental impact categories. The benefits of AD are largely tied to biogas energy recovery, and the fact that, like composting, AD recycles a fraction of nutrients in food waste for subsequent agricultural use.
Anaerobic digestion has the highest eutrophication potential among the food waste recycling and disposal options considered, which is a concern for WWTFs with tight nutrient-based permit requirements. However, surplus electricity production from the CHP system has the potential to be used for additional nutrient removal, thereby reducing this impact. Future studies could explore this management option.
Both WTE combustion and landfilling also capture a fraction of the energy contained in food waste, helping their environmental performance in many environmental results categories. WTE combustion has the second best performance among the included treatment and disposal options. In the absence of this energy benefit, composting struggles to demonstrate competitive environmental performance. However, composting systems do have favorable economic performance, and considerably reduced capital investment costs when compared to AD.
While landfills continue to improve methane capture, results presented here reinforce the perception that there are more resourceful options for leveraging food waste and optimizing environmental performance.
Ben Morelli and Sarah Cashman are with Eastern Research Group, Inc. Cissy Ma and Jay Garland are with the U.S. Environmental Protection Agency (EPA), Office of Research and Development , and Jason Turgeon U.S. EPA Region 1
Disclaimer
This project was developed under Contract No. EP-C-15-010 awarded by the U.S. Environmental Protection Agency. The views expressed in this article are those of the authors and do not necessarily represent the views or policies of the U.S. Environmental Protection Agency. Mention of trade names, products or services does not convey, and should not be interpreted as conveying, official EPA approval, endorsement or recommendation.
Image: Greater Lawrence Sanitary District (GLSD) anaerobic digester, courtesy GLSD