Research shows adding water treatment residuals to a bioswale soil mix immobilizes nutrients in compost that might otherwise leach.
Katrina Mendrey
BioCycle March 2013, Vol. 54, No. 3, p. 36
Bioswales are a green infrastructure tool comprised of an engineered mix of sand and organic matter to support vegetation. The plants and engineered media work to retain and then gradually release storm water during rain events, while also filtering out pollutants in the storm water.
Various standards for exactly what the right recipe is for developing the perfect bioswale soil mix (BSM) for storm water management are being considered or are already in place around the country. These standards vary greatly, but all attempt to address the same challenges — meeting infiltration requirements while providing enough organic matter to support plants and remove groundwater pollutants. The latter is of primary concern as BSM developers try to balance the ability of compost to enhance the filtration qualities of bioswales with the potential risk of contributing phosphorus (P) and nitrogen (N) to downstream waters.
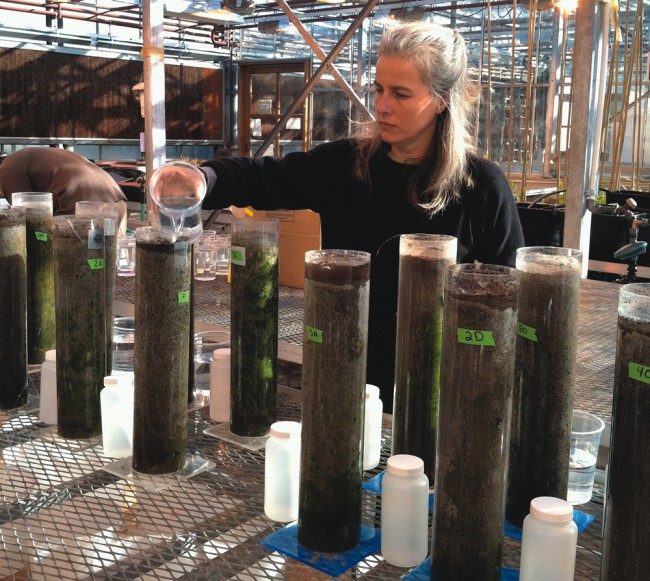
Soils put into the columns were mixed at a base ratio of 40 percent compost to 60 percent silica sand by volume. Targeted PSI levels were achieved before additions of water to mimic two types of storm events.
These standards, while based on good intentions, do not necessarily protect water quality or ensure an optimal storm water management blend. In addition to being a source of nutrients and metals, compost also provides binding for these groundwater contaminants. Other negative impacts of removing the organic element of the BSM are limited plant growth and reduced moisture retention resulting in increased maintenance requirements during dry periods. Faced with such a conundrum one must ask: Isn’t there a better, more consistent way of managing nutrients in BSM?
Phosphorous Saturation Index
An ongoing study at the University of Washington (UW) suggests that there is a better way. Researchers are drawing on established strategies for managing excess phosphorus (P) in agriculture to find a quantitative tool for evaluating different BSMs. Many years of high fertilizer P applications for growing crops have resulted in P saturated soils. Erosion of high P soil to surface water is an environmental hazard. Research in soil science has focused on both immobilizing built up P in agricultural fields and developing tests to measure how saturated the soils are with P. Farmers typically use fertilizers with nitrogen (N), P and potassium (K) combined. As a result, the P is often applied at higher rates than plants demand as fertilizer application rates are based on plant N requirements. Because P is so persistent when bound to soil particles, it leads to a build up of P in the soil. During heavy rain or irrigation events, P can be lost via erosion attached to fine particles or as bioavailable orthophosphate when a soil’s capacity to bind P is surpassed, causing contamination of nearby freshwater through runoff.
Fortunately, P is generally immobile in soils — unless the particles themselves move — and it binds tightly to iron (Fe) and aluminum (Al) hydroxides. In soils high in Fe and Al, P will quickly bind to available sites and remain bound until, in the case of Fe, conditions change such as pH increases above 8 or oxygen levels drop and the system becomes anoxic. This is a rare occurrence in a well-managed field. Aluminum, on the other hand, will continue to bind P across a greater range of soil conditions.
Using this principle, a simple test called the Phosphorus Saturation Index (PSI) has been used to predict mobility of P in fields. The PSI is based on the ratio of P to Fe and Al that is extractable from a soil sample using a solution of ammonium oxalate, a buffer and oxalic acid, a dilute acid. It involves shaking a sample of soil for four hours in the solution, filtering the liquid from the solids, then measuring the amount of atoms, expressed as molar concentration, of P as a ratio to Fe and Al in the solution (Sparks, 1996). The higher the PSI, the higher the probability P will be bioavailable and mobile in the soil, potentially causing eutrophication downstream.
Several studies have indicated that this test can be applied to soils treated with organic amendments, specifically biosolids (Elliot et al. 2002; Maguire et al., 2000 and 2001). Further decreasing the PSI through additions of Fe and Al oxides in the form of Water Treatment Residuals (WTR) has been shown to be an effective method for immobilizing P both in the long-term and for a variety of P sources (Agyin-Birikorang et al., 2007; Elliot et al. 2002; Makris et al., 2005; Novak et al., 2004). WTR, which can be either Al or Fe-based, are often landfilled after use as a flocculent in the drinking water treatment process. However, even after this use they can still have plenty of available sites for binding phosphates and metals, therefore they can be repurposed.
The actual ratio of P to Fe and Al, however, is somewhat situational depending on soil texture and potential for leaching. One suggestion by Elliot, et al. (2002) for a sandy loam soil is a PSI no greater than 1.1. This value is based on results from several leaching studies comparing P losses from a sandy loam soil amended with chicken manure, biosolids or Triple Superphosphate. Results indicated that PSI values <1.1 resulted in P losses no greater than the control.
If a similar method can be applied to BSMs, engineered soil producers such as compost manufacturers can use the simple oxalate extraction previously described to test the PSI of their BSM products. If ratios are found to be high, the recipe can be adjusted with WTR to bind excess P. This may also enhance pollutant removal efficiency of toxins, such as metals, as WTR contain charged sites for chemical binding of both cations and anions. Overall application of the PSI method will allow BSM products to be tested for consistency, addressing concerns regarding nutrient variability between composts — thus expanding the types of compost that can be used in BSM.
PSI Ranges Using Compost In Bioswales
Preliminary results from our UW research suggest that this WTR strategy just might be the answer. The study is designed to determine a PSI appropriate for bioswales, as well as determine if this value can be applied to all composts regardless of feedstock. Further research is investigating whether additions of WTR enhance filtration of metals and nitrate (NO3), and if such additions have any impact on plant growth and storm water infiltration rates.
A range of PSI values (0.1, 0.5 and 1.0) as well as three composts representing yard trimmings, manure and biosolids were chosen as the basis for treatments. Iron-based WTR from a facility in Anacortes, Washington was selected as an additional amendment to reduce the PSI. (As noted earlier, WTR can be either Al or Fe-based.)
Soils were mixed at a base ratio of 40 percent compost and 60 percent silica sand by volume. As the PSIs of the different mixes were all below 0.5, ammonium phosphate was added to increase the PSI with additional P for the 0.5 and 1.0 PSI treatments. WTR was added to all composts to reduce PSI values to 0.1. In the case of WTR, compost volume was reduced to 30 to 35 percent to accommodate for 5 to 10 percent WTR additions based on dry volume. WTR were softened with water to allow for even mixing throughout soil mixes. Tables 1 and 2 display the original PSIs of the individual components and of the mixes prior to and after adjustments with ammonium phosphate and WTR.
Soils were then packed in columns to a depth of 18-inches, the minimum depth specified by the Seattle Public Utilities Standards for bioswales. Columns were leached every 2 weeks over a 12-week period for a total of six leaching events. Two leaching amounts were determined based on the amount of storm water that would be retained by a bioswale of comparable size to each column in a 24-hour/10-week frequency storm (900 ml) and a 24 hour/1-year frequency storm (1,600 ml). Each storm event occurred three times in random order. Influent was a mixture of tap water spiked with 1 ppm copper (Cu), zinc (Zn) and P as well as 2 ppm N — concentrations more than double what is found in typical storm water.
Columns were allowed to drain for four hours prior to sample collection. Collected leachate was analyzed for total and dissolved P, Cu and Zn, as well as NO3-, ammonium (NH4+) and turbidity. Preliminary results from six leachings showed a strong correlation between PSI and dissolved and total P in leachate (Figure 1) as well as some removal of NO3-. While metal removal was seen for all treatments, there was enhanced filtration of Cu and Zn based on lower PSI values (Figure 2 and 3). Further PSI also seemed to indicate reduced turbidity of leachate (Figure 4).
These results were consistent between composts, suggesting that this method can be applied to all composts regardless of feedstock. Next steps include extending the study to look at effects on plant growth and infiltration rate. Additional leaching tests will also be conducted.
Simple, Effective Tool
These initial results indicate that the PSI is a simple, quantitative method for setting BSM standards. Use of this method may ensure that compost-based soil mixes do not contribute to nutrient leaching while allowing for enough compost in the mixes to support plant growth. This adjustment to BSM standards could provide a useful tool for developing mixes that can be guaranteed to prevent nutrient leaching while also meeting the other requirements of a well-designed bioswale. Additionally, allowing mixes to include other feedstocks and WTR also opens up new markets for local and recycled resources.
Equally important, compost manufacturers would have more flexibility regarding the feedstocks processed and high value end markets for their products. Standards that limit the types of feedstocks used in BSM make it less efficient for producers to both accept restricted feedstocks and make blends that don’t use them. The key is ensuring that the soil mixes have the right PSI for storm water management applications.
Katrina Mendrey is a master’s student working with Dr. Sally Brown at the University of Washington’s School of Environmental and Forest Sciences. She earned her B.A. in Political Science at the University of Montana. After graduation she hopes to continue working in the field of soil science research.