One of the great public health triumphs of the last century — wastewater treatment — is poised for transformation into a community sustainability centerpiece.
Sally Brown
BioCycle March 2012, Vol. 53, No. 3, p. 41
Protection of public health is a laudable goal. Our wastewater infrastructure has been constructed with that goal as the prime directive for the public officials and engineers who have built and maintained these systems. This infrastructure has been amazingly effective. The number of children in societies with wastewater treatment infrastructure who die from illnesses related to water borne disease is about zero. The number of reported cases of cholera is about zero. This is one of the great public health triumphs of the last century.
How did this triumph take place? About the middle of the 1800s, the connection was made between dirty water and disease. A cholera outbreak in London prompted the discovery. Drinking wells became contaminated with water from sewers and people started getting sick. From that point, strategies were developed to move dirty water away from sources of clean water as quickly as possible. And thus began construction of sewer systems to carry this water away, starting with black water (water from toilets), then grey water (water from sinks, showers and now washing machines) and storm water. Most of this dirty water was fed via the path of least resistance (gravity flow) to lakes and rivers where it could mix with the other water and seemingly disappear.
While this approach worked pretty well to protect public health from diseases like cholera, it was actually causing a substantial amount of damage to the environment. Feeding this water to lakes and streams was more than they could handle. The problem wasn’t too much metals or terrible toxic pollution (though there was some of that for sure). Rather it was too much food, too many nutrients. Adding this much extra carbon, nitrogen and phosphorus to lakes and streams caused algal blooms as aquatic organisms chowed down on the new taste treats, depleting oxygen and causing fish kills. These nutrients that can build soils and help to grow plants proved to be too fertile for aquatic systems. (Much the same way we are now coming to understand that the endocrine disrupting compounds that harm aquatic species are generally decomposed in soils.) In Seattle, direct discharge of primary effluent (water with only minimal treatment) into Lake Washington made it look like a mud puddle and stink. Swimming in the lake was not an option. In addition, those disease-causing pathogens that were responsible for this whole infrastructure in the first place were also discharged into the lake.
The Clean Water Act in the early 1970s made great strides to rectify the problem. The overarching goal of this legislation was to make all waters fishable, swimmable and drinkable. I’m a huge fan of the swimmable part of that and now regularly swim distances in Lake Washington. Monies were made available to build or upgrade treatment plants to clean the water before it was discharged into the lakes and rivers. Instead of just letting stuff sink (primary treatment) and then discharging the water, plants were upgraded to include secondary treatment — bubbling air through the water to essentially encourage a microbial eating contest. Essentially, the microbes gorge themselves and then pass out, sinking to the bottom where they get carried away and, in many cases, put into anaerobic digesters.
This secondary process, treatment via aerobic digestion, generally consumes about half of the total energy used during wastewater treatment. And wastewater treatment is often the single greatest energy user in municipal infrastructures. Aerobic digestion is highly effective at reducing both the food value (biological oxygen demand) and nutrient concentrations (nitrogen and phosphorus) of the water, enabling treatment plants to meet their required discharge limits. It also kills a good number of the pathogens. The water leaving these plants now looks a lot like water before it was used to carry anything at all to the plants. In many cases, with only minimal additional treatment, that water is safe for growing grass, irrigating crops and even making ice cubes.
Resource Conservation Dimension
While these achievements have been terrific and highly successful, I’m about to argue that these systems are missing the boat. We are entering a new era of resource limitations. And the systems that were developed to get water clean with no expense spared need to revisit their mission: Resource conservation has to be added to the mandate as part of the way these systems protect public and environmental health.
Let’s go back to the beginning. The primary catalyst prompting construction of centralized wastewater treatment systems was the disease-causing pathogens in the water. That water also contained carbon and nutrients, but that wasn’t making people sick. By diverting that water away from drinking water sources it kept the pathogens away from the people. By putting it directly into fresh water, it made the water systems sick — not with the pathogens but with all of that carbon and those nutrients (i.e., too much food). Hence the second mandate of protecting environmental health (and meeting discharge limits) by turning that enriched water back into plain water again, getting rid of the carbon and nutrients in whatever way was the most efficacious.
As I’ve discussed numerous times in my monthly column (recently renamed “Connections”), we are running out of phosphorus. Each kilogram of mineral nitrogen takes 4 kg of CO2 to produce. In addition to those macronutrients, these systems also process the broad range of micronutrients required for plant growth (see “Nutrient Café,” January 2012). The organic matter that causes algae to bloom if you add it to lakes is mostly released as CO2. It goes up to the sky during secondary treatment’s microbial eating orgy. Put on a different lens, and it could be viewed as a source of renewable energy and/or a way to bring soils back to health. So how about we start looking at wastewater treatment facilities as renewable resource centers? What would these systems look like and how hard would it be to build them?
Let’s start from the standpoint of energy. Most of the energy consumed by WWTPs is used to reduce the BOD or amount of fixed carbon in the water. This is released through aerobic decomposition. Those engorged microbes that have done all of the eating and passed out are normally taken to anaerobic digestion tanks where they are in turn eaten along with the stuff that sank during primary treatment. This is done to kill pathogens (back to that disease control mandate again) and to reduce volatile solids. Volatile solids are carbon compounds that readily turn into smelly gasses that can attract rodents and other disease-causing vectors like flies. Reduction of pathogens and volatile solids is required before the solids coming out of these systems (biosolids) can be land applied.
Back in the day, and for many plants that day is still right now, the methane produced during anaerobic digestion was a waste material. It was flared because it contained some water and hydrogen sulfide that made it much fussier to use as an energy source than the power you could get from the grid. I’m happy to say that for more and more plants these days, the methane produced during anaerobic digestion is recognized as a valuable source of energy. And
for more and more plants, it is also being recognized that their anaerobic digesters can make even more energy by adding fresh feedstocks directly to them. Yummy things like chicken blood, used cooking oil, pulped food waste, and even glycerin from biodiesel manufacturing are being added directly to digesters, making lots of methane and generating tip fees for the treatment plants.
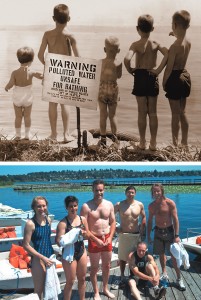
In Seattle, direct discharge of primary effluent into Lake Washington meant swimming in the lake was not an option (above). Today, the author and friends swim in Lake Washington regularly (below). Historic photo courtesy of King County Department of Natural Resources and Parks
Going Further
Increasing energy production is a positive step. Now it is time we figured out how to go a step further. What would it take to transform that secondary eating orgy that wastes all of that energy and uses so much external energy into an anaerobic process? Conventional engineers would normally say ‘no way, the water is too dilute to treat with anaerobic systems’. A recent article suggests however, that this is a real possibility. McCarty et al. (see “Domestic Wastewater Treatment As A Net Energy Producer — Can This Be Achieved?,” Environmental Science & Technology, 2011) contends that a number of systems for anaerobic digestion could be redesigned to replace existing aerated treatments. This would require higher concentrations of organisms that are able to hang out and eat for longer periods without getting swept away with the flow. Two existing options may prove viable — a variation of an upflow anaerobic sludge blanket (UASB) or a modified membrane bioreactor. The authors go on to argue that this would turn wastewater plants into energy neutral facilities rather than high energy consumers.
The McCarty et al. paper was written without even considering adding chicken blood into the mix. The authors are talking about an energy neutral system without codigestion. With codigestion you could create an energy positive treatment plant. What would make this system even more likely to work? Having higher BOD in the influent, (the water coming into the plant) is an obvious answer. One way to do this is to have kitchen food waste grinders in every sink (though, in a conventional system with secondary treatment, i.e., aeration, this ends up increasing energy use rather than producing energy). Another might be to add a portion of the dirty grease early on in the process. And yet another would involve making tighter systems that don’t let storm water dilute the influent.
Storm water is very dilute and enters the wastewater influent stream primarily via combined sewer and water pipes. A rule was passed in the 1970s to encourage separation of storm water from wastewater via separate storm water pipes and treatment systems. In many places, this goal has been achieved to greater or lesser extents. But not fully, and that is why we still have issues with combined sewer overflows (CSOs) that happen when it rains too much and the storm water goes into the wastewater systems. All the extra water makes these systems exceed their capacity and overflows occur. When this happens you get direct discharge of untreated wastewater and storm water into lakes and streams and even beaches. Just last year in Sydney, Australia I saw signs on the beach that said swimming after heavy rains was not recommended. You may also have seen these signs and now you know what they mean.
How would it be if we expanded bioretention systems and rain gardens to intercept more storm water so that it wouldn’t enter the treatment plants? And how would it be if we used municipal biosolids, the material generated during wastewater treatment, as a key ingredient in bioretention systems? We’ve started working on this at the University of Washington and have seen that mixing biosolids compost with some beauty bark and water treatment residuals (the clays left over after drinking water is filtered) makes for a more effective storm water blend than the traditional yard waste compost. It has higher infiltration rates and is better at filtering metals and nitrogen. So here you would use a by-product of wastewater treatment to reduce flows of storm water into treatment plants, concentrating the flows so that completely anaerobic systems have a higher likelihood to succeed. You can start to see how revisiting these systems can make them more sustainable.
We can also focus on keeping the nutrients rather than trying to eliminate them. Nitrogen is a real headache for wastewater managers. That is because it is such an important nutrient for freshwaters and adding too much can cause blooms. This means that N concentrations in effluent discharges are tightly regulated. Engineers have come up with systems to reduce N content in the water leaving the plants. Denitrification cycles can be mixed in with aerobic cycles during secondary treatment. This makes a lot of the mineral or fertilizer N turn into nitrogen gas and volatilize. It might be possible to keep a majority of this N in mineral or organic form. Increasing the carbon loads for anaerobic digestion, thus increasing the C:N ratio, may help to conserve N. And so may struvite precipitation. Struvite precipitates in pipes in plants and clogs them. It also happens to be an excellent concentrated fertilizer, providing both N and P for plants.
We can go on to talk about using the water at the end of the treatment process for things like irrigation and industry. It turns out that pretty much everything that goes through those plants (with the exception of pathogens) is a resource. And we currently treat most of it as waste.
Making The Transformation Happen
This whole discussion of transforming the mission of wastewater treatment plants — from public health through pathogen destruction and environmental health through cleaning water to public and environmental health through resource recovery — requires a new mindset and a whole lot more. The Clean Water Act of the 1970s came with a price tag. It funded a wide range of treatment plant upgrades and it funded education for civil and environmental engineers to teach them how to build and run these plants.
This next transformation also comes with a price tag, again for plant upgrades and training to run these new facilities. We will also need to fund research to develop fully anaerobic systems. Research to understand how to optimize resource recovery. Research to expand use of both biosolids products and reclaimed water in urban areas. Much of this research is already underway. Pieces of this holistic vision are already being carried out. Just read this magazine.
And when we understand all of the pieces necessary to put this in place, we will also need the regulatory and municipal foresight to make sure that it gets put into place. A regulatory environment willing to work towards these goals must be created. Strict regulations now make sure that treatment plants meet their discharge limits. We will need understanding regulators who encourage plant operators to try these new systems, rather than holding back because of a fear of exceeding discharge limits. Finally, we will need municipal governments and treatment plant operators willing to add another environmental cause to their job description.
Sally Brown is a Research Associate Professor at the University of Washington. She writes a monthly column for BioCycle, and is a member of the magazine’s Editorial Board. Email Dr. Brown at slb@u.washington.edu.